1. X-ray Free Electron Lasers
XFELs Around the World
The worlds´ first XFEL was the SLAC’s Linac Coherent Light Source (LCLS) in the US in 2009. Since then, four more followed (SACLA in Japan, The EuXFEL in Germany, PAL-XFEL in South Korea, and SwissFEL in Switzerland), two more are currently under constructio (LCLS-II in USA, and SHINE in China), and one is on planned (UK-XFEL in UK)

Five XFELs currently available worldwide.
How does an XFEL Work?
X-ray free electron lasers (XFELs) are X-ray light sources of a few Km long (~3Km) capable of producing very intense X-ray pulses of brief duration. XFELs produce electron bunches, which are accelerated to the speed of light in a linear accelerator of a few kilometers long before they reach the undulators....
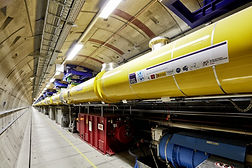

Accelerator tunnel (left) and undulator hall (right) at the EuXFEL (Photo credits: EuXFEL)
....Once there, the electrons wiggle via the magnets allowing the electrons to interact with their emitted radiation, which causes microbunching of the electrons with spacing equal to the wavelength of the emitted X-rays. As the electrons proceed through the undulators, their emission becomes more and more coherent leading to highly coherent pulses with femtosecond duration.....
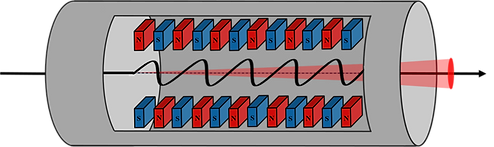
Schematic representation of an undulator segment. Figure from Martin-Garcia et al., 2016. ABB
....The extremely high number of electrons in a micro bunch (on the order of a billion), the high longitudinal coherence, and the ultrashort duration of the pulses, pushes the peak brilliance 10 orders of magnitude higher than that currently achievable at synchrotrons, making XFELs the Worlds’ most powerful X-ray light sources.
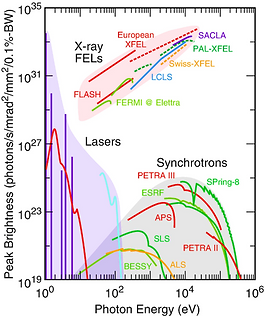
Comparison of peak brightness as a function of photon energy between conventional lasers and higher harmonic generation sources, synchrotron sources, and X-ray free electron lasers. Figure from Boutet and Yabashi. Springer. 2018.
On the right is an educational video produced by the European XFEL where you can see how XFELs work.
Watch it on You Tube here
Credits to EuXFEL for producing this amazing video!
2. Serial Femtosecond Crystallography
The unique properties of XFELs (high brightness and short pulse duration) have facilitated the appearance of a new scientific-technological advance in the field of structural biology, the serial femtosecond crystallography (SFX) (Chapman et al., 2011. Nature)
SFX relies on the “diffraction before destruction” principle in which the photon scattering events by crystals when hit by XFEL pulses occur so rapid than diffraction from crystals can be recorded before being destroyed (Neutze et al., 2000. Nature)

Explosion of T4 lysozyme (white, H; grey, C; blue, N; red, O; yellow, S) when exposed to an X-ray FEL pulse with an FWHM of 2 fs, and disintegration followed in time. Atomic positions in the first two structures (before and after the pulse) are practically identical at this pulse length induced by XFEL pulses. Figure from Neutze et al. Nature. 2000.
In SFX, data sets are no longer collected from single, cryo-cooled crystals mounted on a goniometer; instead, hundreds of thousands of nanocrystals or microcrystals are delivered to the XFEL beam in a serial fashion, in random orientations, at room temperature, so that only one snapshot is recorded per crystal per X-ray pulse. Because the XFEL beam is so intense, crystals must be constantly replenished between X-ray pulses as the crystals are literally destroyed upon each shot. More experimental aspects on this new way of doing crystallography can be found in the following sections below.

Schematic representation of the experimental setup of a typical SFX experiment at XFELs. Randomly oriented nanocrystals (green) in their mother liquor are delivered into the focus of X-ray beam by a gas-focused liquid injector. The X-ray beam, which is transverse to the jet, hits the crystals in the interaction region and diffraction snapshots of single crystals are recorded on a detector. Figure from Martin-Garcia et al. ABB. 2016.
3. SFX with XFELs: Applications in Biomedicine and Biotechnology
Because SFX is conducted at room temperature, it enables not only the structure determination of static structures, but also time-resolved studies to understand conformational dynamics by determining the structure of intermediate states in enzymatic reactions at timescales of 100 fs to minutes. The short duration of pulses produced by XFELs enables SFX to overcome the major limitations of macromolecular crystallography with synchrotrons: radiation damage. Thus, SFX enables studies of macromolecules under “native” conditions at room temperature in the mother liquor, which has greatly impacted various research areas in structural biology:

Crystal of the protease CatB in living insect cells. Figure adapted from Koopman et al., 2012. Nature Methods,

Structure of CatB determined by SFX from the in vivo grown crystals. Figure adapted from Redecke et al., 2013. Science.
In vivo crystallography
In vivo grown crystals have two main limitations by which they are not suitable for X-ray measurements at synchrotron light sources: 1) They tend to be very sensitive to radiation damage; and 2) their size is proved to be too small (a few microns), being typically restricted to the size of the cells. The advent of high-brilliance XFELs and the establishment of SFX have opened new avenues in structural analysis using in vivo grown crystals to determine protein crystal structures.

G protein-coupled receptors (GPCRs)
GPCRs belong to a large family of cell receptors involved in some of the most chronic human pathologies including cancer, cardiovascular diseases, diabetes, obesity, and immune disorders; being, likely, one of the most attractive targets for structure-based drug discovery. However, obtaining large, well-diffracting crystals for data collection at synchrotrons is very challenging. Crystallization of GPCRs typically leads to the formation of micrometer-sized crystals, which make them suitable for SFX experiments. In fact, SFX (along with Cryo-EM) has contributed notably to the rapid growth of structural studies of GPCRs.
Crystal structures of GPCRs determined by SFX from 2013 to 2019. Figure from Fromme et al., 2020. eLS.
Structural Dynamics and Molecular Movies with XFELs
Due to the porous nature of macromolecular crystals with large solvent channels that allows ligands to diffuse into the crystals, along with the small size of the crystals used in SFX (in the nano- or micrometer range), SFX with XFELs offers the possibility to capture functional dynamics by the so called time-resolved experiments (TR-SFX). There are two types of TR-SFX experiments: 1) pump-probe TR-SFX experiments, in which crystals are injected into the path of a pump laser before being probed after a predetermined time delay by the X-rays, and 2) mix-and-inject TR-SFX experiments, where protein microcrystals are mixed with a substrate so that the substrate rapidly diffuses into the crystals and binds to the protein.
Examples of successful TR-SFX experiments are shown below.
Photoactive Yellow Protein (PYP)
The molecular movie of the trans-to-cis isomerization of the photoactive center (a molecule of p-coumaric acid, PCA) of PYP, was “filmed” between 100fs and 3ps. The movie reveals that PYP remains in an excited state for about 600 fs upon light absorption before the chromophore atoms, still in trans configuration, begin to shift and continue over a 3ps time delay where the chromophore is in a cis configuration.
Watch the movie on You Tube here.
References:
1) Tenboer J, et al. 2014. Science. doi:10.1126/science.1259357
2) Pande K, et al. 2016. Science. doi:10.1126/science.aad5081
Beta-Lactamase C (BlaC)
The proof-of-concept of a mixing experiment at XFELs was done for watching, in “real time”, the inactivation of an antibiotic (Ceftriaxone, CEF) by the enzyme BlaC from Mycobacterium tuberculosis. Reaction intermediates were captured by collecting data sets at time points between 5ms to 2s upon mixing the protein crystals with CEF. From these experiments, it has been discovered that CEF binds BlaC shortly after mixing and that the nucleophilic attack by Serine70 and the subsequent opening of the β-lactam ring, occur 500ms later.
Watch the movie on You Tube here.
References:
1) Kupitz C, et al. 2016. Struct Dyn. doi:10.1063/1.4972069
2) Olmos, J.L., et al. 2018. BMC Biol. doi: 10.1186/s12915-018-0524-5
3) Pandey S, et al. 2021. IUCrJ. https://doi.org/10.1107/S2052252521008125
Bateriorhodopsin (bR)
The molecular movie of the photocycle of bR has been constructed at time delays from femtosecond to millisecond regimes. From these experiments the intermediates involved in the isomerization of the retinal molecule, the structural rearrangements undergone by bR, as well as the water molecule network acting as a proton wire, were all captured as a function of time.
Watch the movie on You Tube here.
References:
1) Nango E, et al. 2016. Science. doi:10.1126/science.aah3497
2) Nogly P, et al. 2018. Science. doi:10.1126/science.aat0094
3) Weinert T, et al. 2019. Science. doi:10.1126/science.aaw8634
Adenine Riboswitch
The reaction of a riboswitch molecule with its adenine substrate was followed for a time delay of 10min. During the first 10 seconds, the adenine molecule diffused into the crystals, bound and reacted with the RNA molecule, being able to capture the full mechanism. Then, if the reaction is left to evolve, between 10s and 10 min, a polymorphic transition occurs inside the crystals triggered by the substrate. Riboswitch molecules, initially in a P21 space group, underwent huge conformation changes leading to a P212121 space group.
Watch the movie on You Tube here.
References:
1) Stagno JR, et al. 2017. Nature. doi:10.1038/nature20599
2) Stagno JR, et al. 2017. FEBS J. doi:10.1111/febs.14110
To find more on Structural biology and SFX at XFELs please visit the CBE website here